Mendel Part 2: Breaking the law(s)
- Jack Marsden
- Jan 22, 2021
- 6 min read
This is part 2/2 in a brief series on Mendelian genetics. If you haven’t read part 1, that can be accessed here. It describes Mendel’s famous pea experiments and outlines his laws of inheritance. If you’ve read part 1, or if you’re pretty confident with how simple Mendelian genetics works, please read on.
This article describes what Mendel was wrong about. Of course, throughout his life he was probably wrong about a lot of things, as are we all, but we will focus on what he was wrong about with regards to his laws of inheritance. But first, I’ll describe a second, less well known experiment that Mendel conducted after his pea experiments.
Hieracum, apomixis, and the unravelling
Mendel’s only other published paper on inheritance was an attempt at replicating his wildly successful pea experiments in another organism. This organism was another plant, the hawkweed (genus Hieracum). This paper was published in 1870, and appeared to destroy all of his hard work on pea plants. No matter how much Mendel crossed the hawkweed plants, they all bred true, with no variance in offspring at all. Whereas Mendel’s lovely 3:1 ratios existed in peas, hawkweeds bred at 4:0. It’s hard to imagine what effect this had on Mendel and how it made him view his original experiments, but it must have been quite demoralising. That said, we still teach Mendelian genetics today, so how could he have been so very, very wrong?
The answer is simple: apomixis. Well, the word isn’t simple, but what it describes is. Apomixis is a method of producing seeds where all genetic material comes from the maternal parent. If humans gave birth by apomixis, every child would be a clone of their mother. As was discovered in the early 20th century, hawkweeds produce seeds via apomixis. So of course there wasn’t any variance in the offspring: he was essentially cloning the maternal plants ad nauseum, not recombining the genetic materials of two parents. It was, to put it mildly, a very unfortunate choice in organism on which to conduct follow up experiments. Now, on to the laws.
Mendel’s laws: Then and now
Each of Mendel’s laws (of which I’ve decided there are four, though as noted in part 1 of this series, it’s hard to get a consistent line on this) is very useful in its own ways, and together perfectly describe Mendel’s pea experiment. Though, as is hardly surprising, they are incomplete, and viewing genetics solely through the lens of four principles derived by an Austrian monk 150 years ago will not give you a very full understanding of genetic science compared to our current understanding. So, how should we augment these laws and what are some known exceptions?
Law 1: the law of paired factors
This is a pretty robust one. The current terminology for an organism with two copies of each gene is a diploid organism, and these dominate our world: humans, mice, worms, flies, most organisms we study closely in genetics are diploid organisms, and conform almost perfectly to this law.
One exception is polyploid organisms: some organisms have four sets of genes (tetraploidy), some have five (pentaploidy), and some have all the way up to twelve sets of genes. The reasons for this are a little complex to bring up here but I’ll write about it on its own sometime. There are examples in humans too, most famously downsyndrome, which arises from having three copies of chromosome 21. This originates from errors in the creation of sex cells, most commonly in the female parent.
The other major exception to this is sex chromosomes. Whilst (in humans) females have two copies of every gene (because they have two X chromosomes), males only have one copy of certain genes on the X and Y chromosomes. A technical way of expressing this is saying that female humans are homogametic and male humans are heterogametic. In some species, such as birds, females are heterogametic whereas males are homogametic. In many organisms, sex is not determined by chromosomes at all. Sex determination in humans and other organisms is another topic far too complex to dive into in the detail it deserves here and will also be a topic of a future article.
Law 2: the law of dominance
This law is a little less robust. Mendel was very fortunate choosing traits with two possible genes which had very simple dominance relationships. In our example from part 1, the two potential traits were yellow and green seeds and yellow seeds were dominant to green seeds. This does occur in nature, but is far from the norm. In most genes, there are multiple, often hundreds, of possible alleles of a gene*. Moreover, sometimes there is a simple dominance relationship, as in peas, but often there is codominance (the traits of both alleles are expressed) or incomplete dominance (a combination of the two alleles is expressed.
Moreover, most physical traits aren’t just affected by a single gene. They’re affected by the interactions between numerous, sometimes hundreds, of genes, especially more complex traits such as height. Height, unlike seed colour, exists on a spectrum: you’re not either 5 feet tall or 6 feet tall, human height, your height is somewhere on a huge range of human heights, and is affected by around 700 different genes.**
So, dominance is a neat concept that is very helpful in some real situations, but is usually unhelpful when thinking about real genetics.
Law 3: The law of segregation
This one is fairly spot on. The only situations where this falls down are in situations such as downsyndrome wherein one parent gives the child two sets of a chromosome and the other parent contributes only one. This is a bit of an anomaly, and anomalies always exist, but other than anomalies such as this the law of segregation is probably the strongest of Mendel’s laws, and his legacy.
Law 4: The law of independent assortment
This one is a little tricky. Many genes are inherited independently of each other, but many genes are not. Certain genes are inherited more frequently together than apart, as was discovered in the Cold Spring Harbor Laboratory by TH Morgan, AH Sturtevant and others in the early 20th century. The reason for this, as we know now, is proximity. Generally speaking, the closer two genes are on a chromosome, the more likely they are to be inherited together. Once they’re a certain distance apart, the assortment is indeed independent. If the genes lie on separate chromosomes, the assortment is independent.
Hold on a second, if the genes are on the same chromosome, shouldn’t they always be inherited together? When sex cells are made, don’t you get a whole copy of each chromosome in each sex cell? No, and yes. The thing is, when sex cells are being made, the chromosomes themselves recombine. For instance, in a cell dividing to create two sex cells (this kind of cell division is called meiosis), the two different copies of chromosome 1 in that cell swap certain parts to create two new chromosomes, and these chromosomes are then given to the resulting sex cells. Each one of these chromosomes is distinct from either of the original chromosomes in the original cell. This act of recombination is known as crossing over, and is very poorly demonstrated in the below diagram.
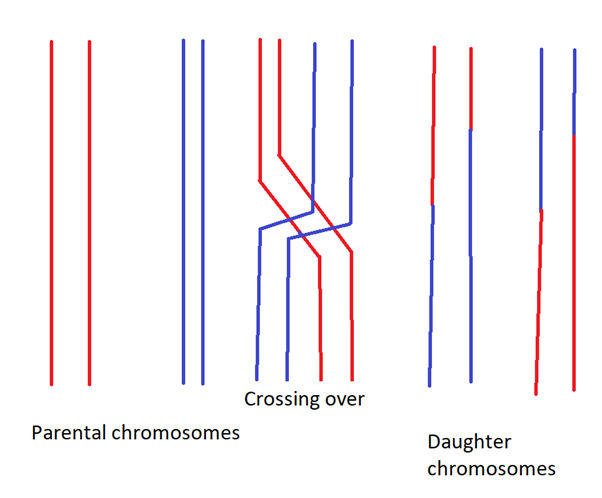
You bet if I ever make any money from this, the first thing I'm spending it on is a graphic designer
Crossing over occurs at more or less random places (known as chiasma, plural chiasmata) along chromosomes,*** and usually happens 2-3 times in animal chromosomes. It has to happen at least once per chromosome, or there are problems with sex cell creation (it is often a fail to cross over that leads to downsyndrome). Crossing over creates huge amounts of genetic diversity, which is important for the long term evolutionary fitness of an organism.
This is all to say, the closer two genes lie together on a chromosome, the less likely it is they will be separated by a chiasma, but if they are far enough apart, there becomes a 50% chance there will be a chiasma between them, and assortment becomes independent once again.
Thus concludes the pair of articles on Mendel. As a scientist, his work is arguably unparalleled in how it influenced the way we understand genetics, and appreciating his contribution as well as acknowledging its shortcomings is essential to having a full understanding of modern genetics.
I hope you learnt something new!
*An allele is a specific type of gene. Yellow seeds and green seeds are two alleles of a gene at a certain place (a locus).
**That are known. There may be more.
***Certain regions on the chromosome are known to cross over less, but this is unimportant for the level of understanding we’re aiming for here.
Commenti